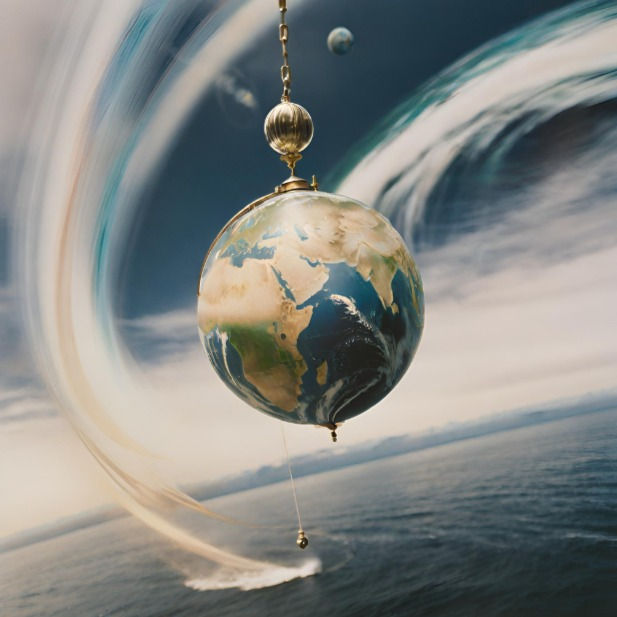
As a first play with python on a mobile app, I was impressed by the seemless install, ease of coding, excution and neat graphical interface.
Coding the dynamics of the double pendulum as an example to test imports of integration and graphing modules, it was apparent the coupled system provides a good analogue for the climate "chaos" (sorry, "transition" for a less dramatic take) that may follow the tipping points that are now talked about as part of the climate emergency.
These "tipping points" commonly arise in systems that are coupled. In the case of the double pendulum, the coupling is clear, one pendulum hangs from the base of a another and forces from the motion of one pendulum under gravity, evidently affects the other. Detail one this later, first to the key points:
Climate stability emerges from a complex "dance" between coupled Earth systems, the atmsphere and oceans playing the prima ballerinas
The dance is fueled by energy in the atmosphere and oceans from solar radiation and the radiative forcing associated with natural and now dominant man-made processes
The AMOC, is an ocean current system that is strongly coupled to the atmosphere through heat exchange and evidence suggests it is slowing and heading for a tipping point!
The double pendulum provides a simple, visual analogue to understand the complex dynamics of these systems and their tipping points ... also fun to play with!
Here we light touch on the physical drivers of these coupled systems, their senstivity to (not such hard) knocks, changes in the "dance" as they approach tipping points and the outcomes from tripping over the point of no return.
Dancing to the tune of the sun, sea and sky
Of course, the climate is way more complex than two flip-flopping pendula, but it's behaviour is strongly regulated by the coupling of two dominant systems, the atmosphere and oceans. The average ocean depth (3.7 km) and effective height (scale height) of the atmosphere (8.7 km) are much less than the radius of the Earth, so the relative "size" of interacting land, sea and sky follows from their respective surface coverage, 30%, 70% and 100%. [1]
The interaction between the atmosphere and ocean is crucial to maintaining balance in the Earth's climate, with profound implications for weather patterns, ocean circulation, and climate stability when the system is kicked out of whack!
Many factors feed the dynamics of the atmosphere and oceans. The main driver, the sun, supplies energy at a rate of 340 W/m2, but natural greenhouse gas (GHG) emissions from volcanoes, ocean vents and biomass degrdation, to the albedo of polar and glacial ice, clouds and aerosols modulate the balance of incoming solar and outgoing (blackbody) radiation of the planet.
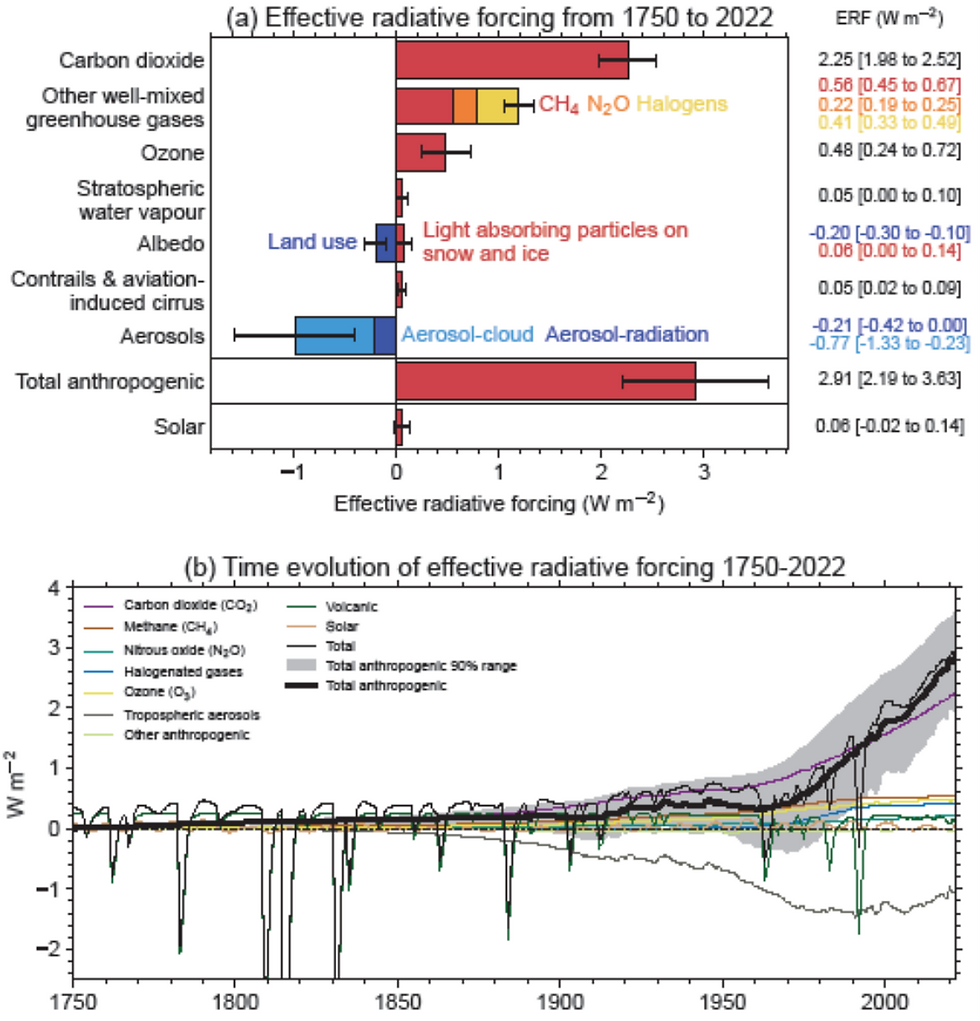
Known as radiative forcing, anthropogenic GHGs now adds a near 3 W/m2 [Fig (a)] to the solar irradiance, from IR emitting molecules CO2, CH4, N2O and CFCs [2]. A shocking 100% increase in forcing from 2005 (1.5 W/m2), when I was teaching this stuff to undergrads!
Net radiative forcing has increased steadily since the industrial revolution around 1750 [Fig (b)], with rising atmospheric CO2 from burning coal and gas, but a sharp increase following a post WWII rebuild boom has led to the soaring global temperatures we now experience in the 21 century.
Temperature and forcing link through the Stefan-Boltzmann (SB) equation, which relates the irradiance (W/m2) of a body to the fourth power of its temperature (via the SB constant 𝜎 = 5.67 x 10(-8) W/m2.K4). A positive radiative forcing (∆E) is just the difference between the SB eqn at temperatures before (Tb) and after (Ta) warming of the body (Earth).
The final temperature is a function of the radiative forcing, which between 1750-1960 shows a slow linear increase from around 0.2 W/m2 to 0.3 W/m2 over the 210 yr period, but after becomes a much steeper rise to 3 W/m2 over 60 yrs to 2020.
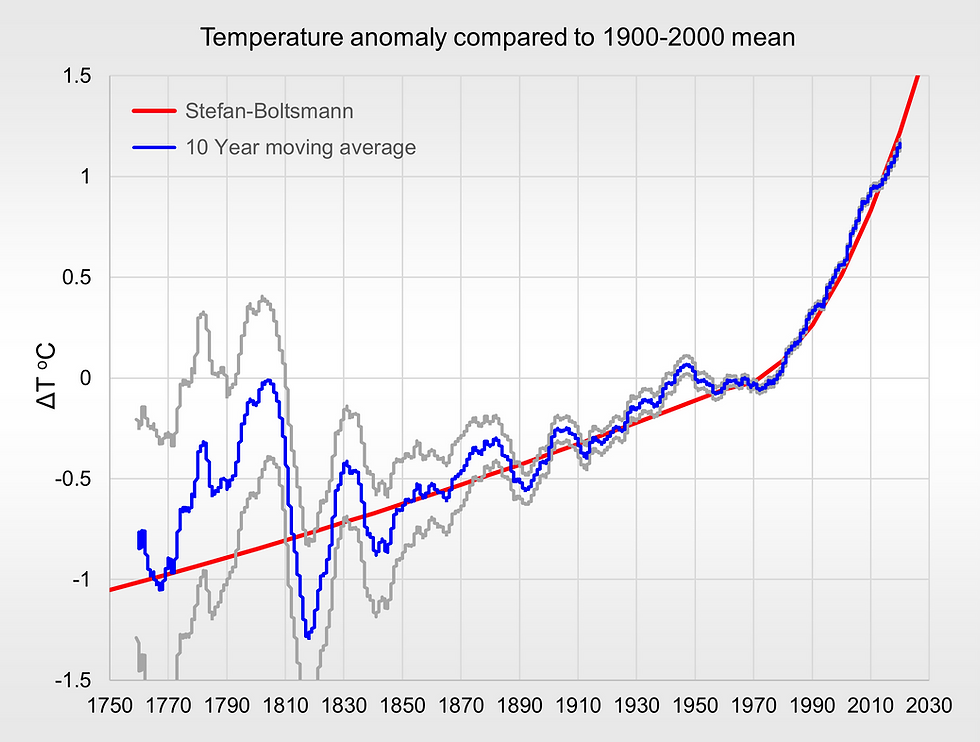
Plugging lines representing the two gradients in radiative forcing into the SB eqn, reproduces the warming trend since 1750 [3] and the famous "hockey stick" graph, coined in the early noughties to describe the seminal analysis of global temperatures over 1000 years by Mann et al [4].
But global averages disguise a crucial difference in the reponse time of the atmopshere and ocean to radiative forcing. This arises from large differences in their heat capacity (kJ/m3.K) and thermal conductivity (W/m.K).
What the heck are these? The first, the energy required to heat a volume of material by a degree Kelvin (K); second, the rate at which energy moves through the material for every degree difference in the temperature gradient across a metre length.
The heat capacity of water (4 MJ/m3.K) is some 4000X that of air (1 kJ/m3.K), and thermal conductivity (0.6 W/m.K) is 24X that of air (0.025 W/m.K). Why? Think density, water as a liquid has more molecules per m3, over which to distribute any energy input, and a smaller (kinetic) energy per molecule corresponds to a smaller temperature rise. But equally, more frequent collisions between molecules, transfers energy more rapidly.
So what? It means the oceans have been a buffer to more extreme warming than currently observed; a heat sponge that quickly removes energy from the warming atmosphere, with a large capacity to store the energy through a slower heating per Joule absorbed.
NASA/Goddard Space Flight Center Scientific Visualization Studio [5]
Nonetheless ocean waters expand on warming, leading to sea level rise. More importantly changes in temperature gradients between a warming sea surface in contact with the atmosphere and cooler deep waters lead to a significant impact on the ocean currents that regulate climate through feedback.
Currents such as the Atlantic Meridonal Overturning Circulation (AMOC), deliver warm tropical waters to cold polar regions, where surface currents warm the contacting atmosphere, while deep currents return cooler water to the tropics.
Cycles, kicks and choas
To the point! the atmosphere and ocean are just one pendulum coupled to another, albeit the forces that keep them moving are way more complex than a simple nudge of the bob! But what happens when the system is pushed out of whack?
The double pendulum provides a simple and insightful analogue. First a bit of maths, but skip if you don't see the beauty.
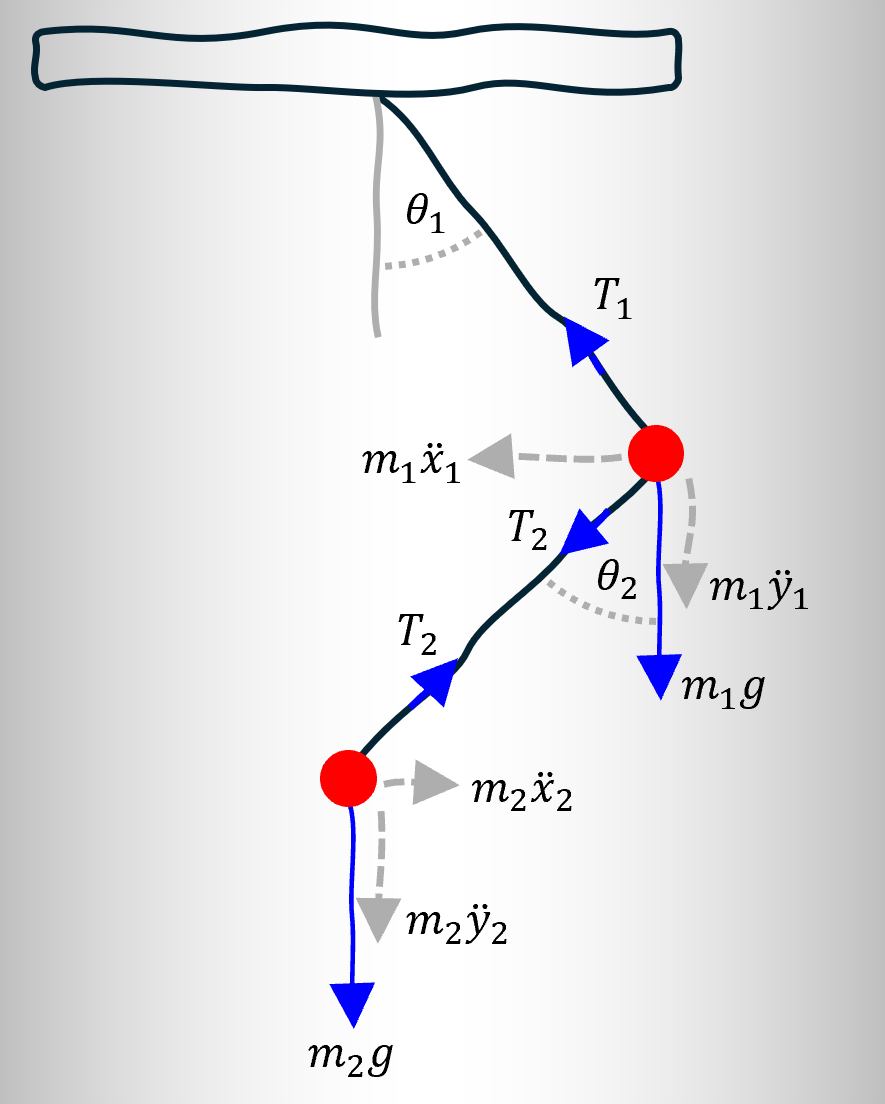
Formally, it's not only gravity that acts on the pendulum, but also tensile forces that keep the pendula connected together and to the support. These can all be resolved into orthogonal forces for each pendulum, given by the product of mass and accelerations along each axis (x, y):
With some lengthy algebra the Cartesian components can be expressed as the rotational acceleration (rate of change of angular velocity) of each pendulum [6]. To integrate the swing of each, the second order differentials in their angular displacements are reduced to first order in the angular velocity:
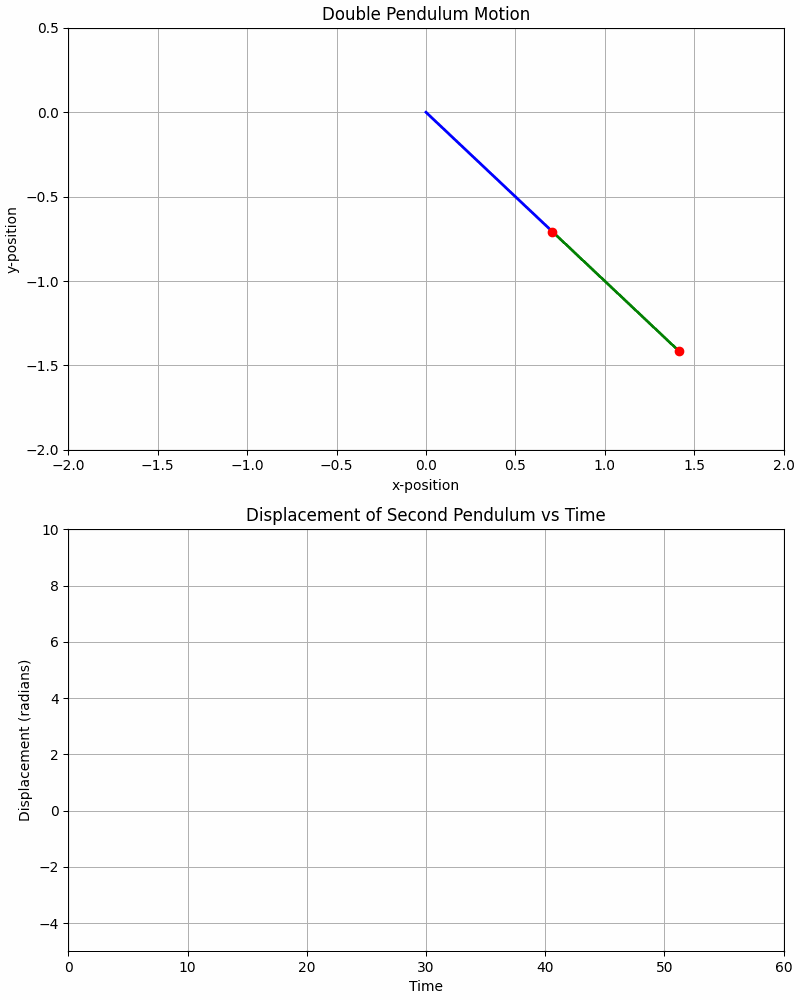
Here python and the handy import scipy do the hard work of integrating the rate equations to give trajectories of the angular velocities, from which the components of displacement can be resolved for plotting a nice graphic.
Animations show the flip-flop of the double pendulum (top) and the radial displacement of the lower pendulum over time (bottom).
And how does this relate? Imagine the upper pendulum represents the atmosphere, the lower, the ocean. For the first 20 seconds (think past 20000 years or so), the atmosphere and ocean remain in sync with the systems happily going through their natural cycles of circulation and exchange. Oscillations might represent the 11 year sunspot cycle [7], for example, or longer cycles associated with Earth's orbit, tilt and rotation [8].
Then the industrial revolution hits in 1750 and kicks the atmosphere with an injection of anthrogenic CO2 (and other GHGs) over 270+ years to present day; a short, sharp, shock to the system on the timescale of climate cycles.
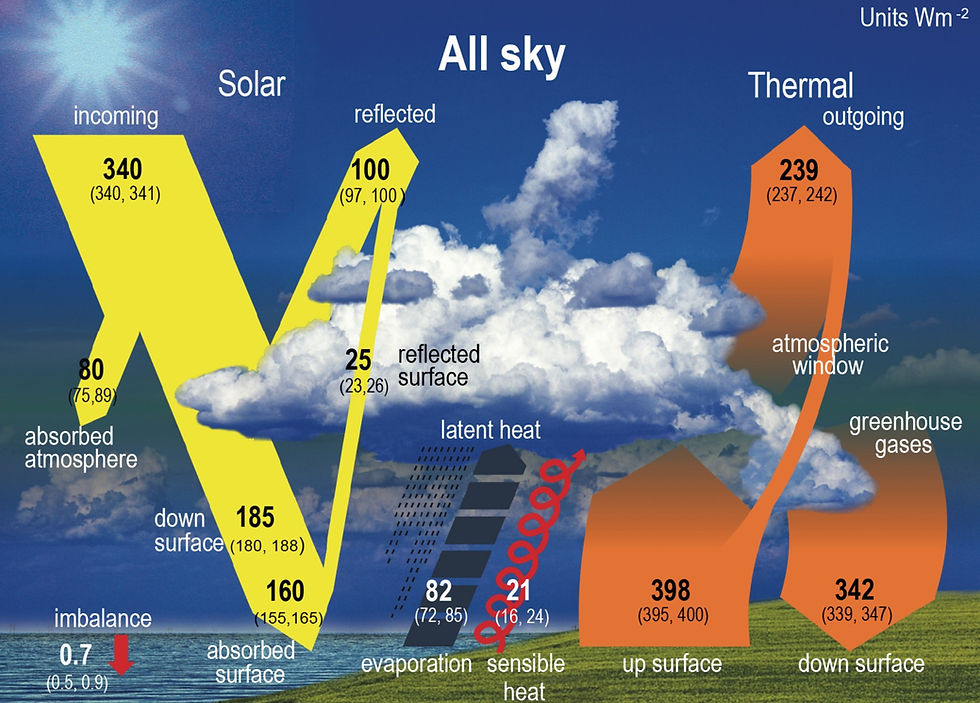
Levels increase by over 50% from 280 ppm (1750) to 420+ ppm CO2 (2024) [9], trapping and re-emitting around 86% of the 399 W/m2 of IR radiation emitted from the Earth. The 343 W/m2 returning to the surface then gives the net positive 3 W/m2 radiative forcing that adds to the solar irradiance, and which is now leading to pressure on Earth's regulatory sytsems.
The result? Well we see our "ocean" pendulum, exhibiting more unstable, punctuated swings after the kick to the "atmospheric" pendulum, followed by a large transition (+2π rotation) around 35 s, to a more chaotic state that settles to swings and cycles of greater amplitude. Great, but what observations in the atmosphere-ocean dynamics might this mimic?
The atmosphere and oceans have undergone large transitions over many geological timescales, eras (100M yrs), periods (10M yrs), epochs (1M yrs) and ages (10K-100K yrs), with variable duratons of warming and ice ages.
These are driven by the Milankovitch cycles [11], changes in Earth's orbit (100-400K yrs), tilt (41K yrs) and pressession (26K yrs) with respect to the sun, which affect levels of solar forcing across different regions of the Earth, as the longer and shorter cycles move in and out of phase (check the excellent detail on this at Carbon Brief [8]).
Cycles on shorter timescales (2k-5K yrs), known as Dansgaard–Oeschger [12] events are less well understood, but thought to follow a chain of changes in atmospheric circulation, regional warming and increased ice melt and/or precipitation; ending in freshwater forcing on the AMOC and cooling due to changes in its circulation of heat in the northern hemisphere.
There is a strong correlation between warming and CO2 levels during these periods, with global temperature rises lagging the GHG increases. Although there is some uncertainty whether the lag is flipped in Southern hemisphere [8].
In short, sea levels drop when ice sheets extend during ice ages, land is exposed, natural reforestation increases atmospheric CO2, and temperatures finally follow from an accumulating greenhouse effect.
When the AMOC runs amok
However, recent measurements and historic proxies for CO2, show ppm of the gas has never been higher in the last 800,000 yrs than it is today and temperature anomalies are now approaching, if not exceeding, the IPCC/COP 1.5 Celsius marker.
In fairness to other coupled Earth systems, it should be noted that kicks to the atmosphere don't just affect the oceans and other tipping points are available [13]!

Indeed, the accelerating antarctic ice melt may have already passed the point of no return [14] and this too will add to sea level rise and changes in ocean currents. And that's the beauty of Gaia, everything's connected!
But the ocean is the largest system coupled to the atmosphere, and the AMOC is partcularly interesting because of the feedback it has on the atmosphere from the heat it circulates and exchanges.
The overturning circulation is measured in a unit of the Sverdrup (new to me, ergo cool), where 1 Sv = 1 million m3/s. 15-20 Sv are typically overturned in the north atlantic; that's a lot of warm, saltwater flowing from the south and deep freshwater circulating back from the north. But indicators point to a slowing of the AMOC, having declined by around 3 Sv over a decade of measurements made (26.5 degrees N) from 2004 [15].
Further proxies, such as sea surface temperatures, salinity, marine productivity and sedimentation have all shown declines that correlate with a collapse of the AMOC over the last century or so [16], compared to relatively steady indicators for the last 1000 yrs.
However, large seasonal and decadal variability of overturn rates (up to 6 Sv) and sea surface temperatures as proxies, add uncertainty to observed trends in the AMOC, and whether declines are anthropogenic in origin [15, 17].
Models (CMIP [18]; CESM [19]) also play an important role in assessing AMOC status, strength of coupling to the atmosphere and its role in regulating the climate. But these can be subject to asssumptions, approximations and simplifications that raise questions about their predictive power when they struggle to reproduce past patterns [20].
Latest results suggest that a decline in freshwater transport towards a mininum (at 34 oS), along with its increased variability, is a strong indicator of an approaching tipping point in the AMOC [21].
Back to the double pendulum and hey presto, we see it (above), increased modulation and a "minimum" in the cycle of our lower, "ocean" pendulum (OK, technically a maximum displacement), just before transition to a different swing state!
Stop! Think we've squeezed enough out of the analogy. A different swing at the start, a different strength kick, timing of the kick, mass and length of the pendula, all lead to different outcomes.
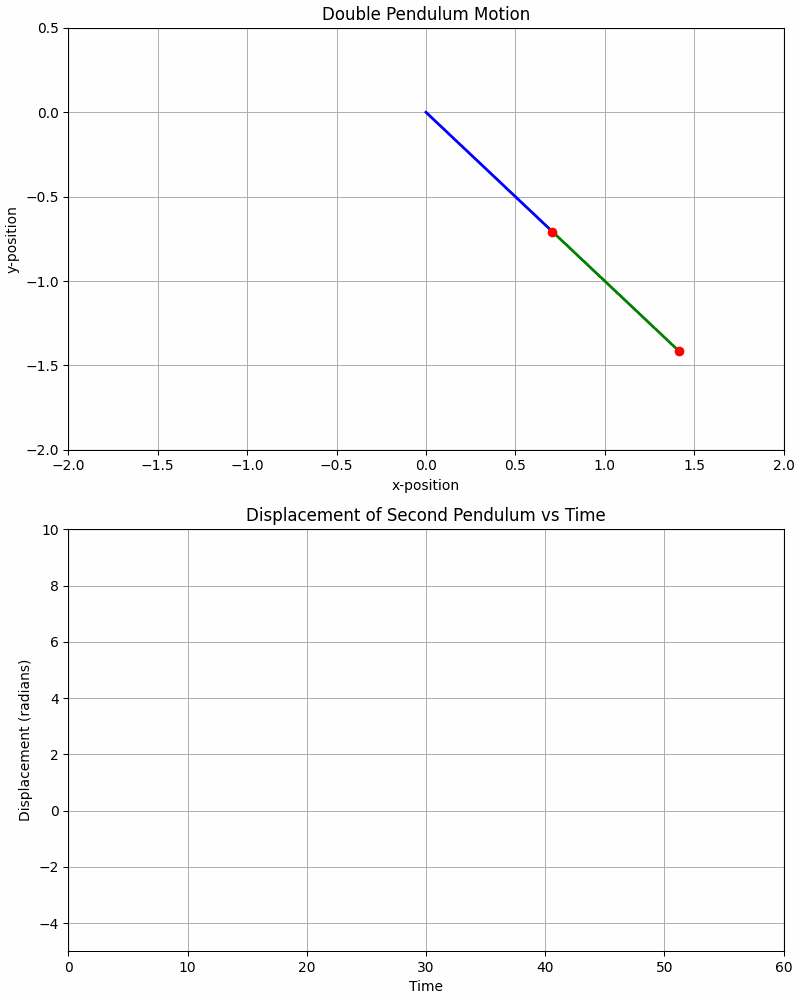
A slightly bigger kick, all else equal, might be expected to turn the pendulum chaotic quicker, but as seen (left), while a different pattern of flip-flopping emerges, the "ocean" does not undergo a full rotation and transition to a second state, at least within the same observation window.
The point here? Even for the simple double pendulum with few variables, the complexity of its motion in the future is exquisitely sensitive to its dynamics in the past, and the size and timing of any perturbation to that motion.
Now imagine the atmosphere and ocean, with many more variables and many more kicks, big and small. Uncertainties in predicting tipping points from models and measurements become very large.
But when models and measurements start to tell the same story, we should to take heed, a tipping point will happen sooner or later, as historical proxies indicate. The question of when is uncertain; the IPCC predict with "medium confidence" the AMOC will not experience abrupt collapse this century [22], others predict as early as 2025 [23], some support this possibility [21, 24, 25], others question it [20, 25].
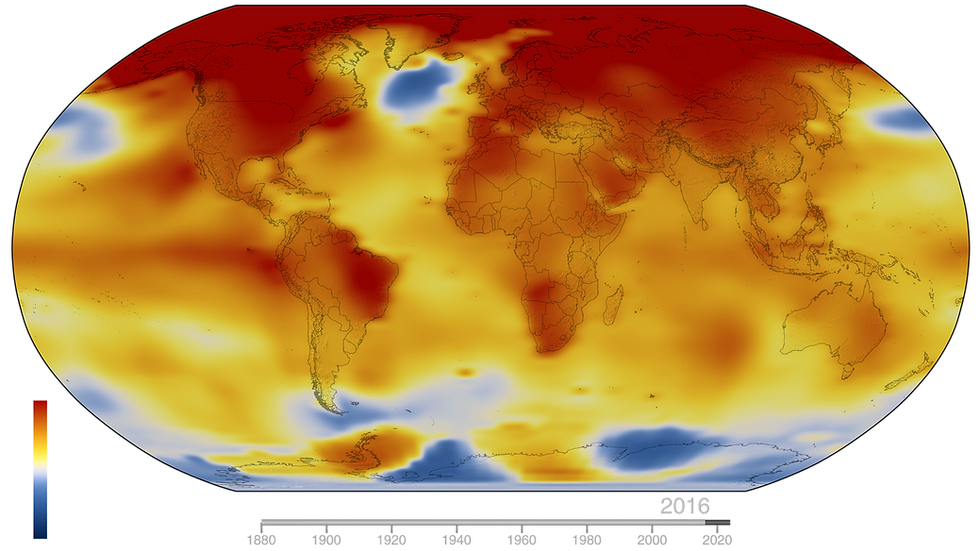
The outcome may be more predictable. The emergence of a "cold blob" (2016) over the North Atlantic [26], has been attributed to a slowing AMOC [27] and the North Atlantic Oscillation (NAO) weather system [28], and connected to harsh winters across 2009-11 [29] and, counter intuitively, recent, higher than average, temperature rises experienced in Europe and the polar Arctic [30].
Following AMOC shut down (2 to 4 Sv), without heat transfer from the tropics, Europe could see temperatures plunging -3 to -10 oC over the seasons, within 50 to 100 yrs of collapse. Southern regions warm slowly without heat flow out of the hemisphere and precipitation is predicted to increase over the tropics, flipping the wet and dry seasons over the Amazon [22, 31].
While more recently, "blob" temperatures appear to recover toward the northern latitude, anomaly norms, debate around AMOC tipping and its consequences has clearly grabbed the attention of mainstream media. With a healthy mix of dramatic headlines [32], sceptical opinion [33] and more measured commentary [34], the parley over whether "The Day After Tomorrow" [35] is actaully "Two Days Before The Day After Tomorrow" [36], or far in the future, will rumble on.
Back to some fun with the double pendulum...
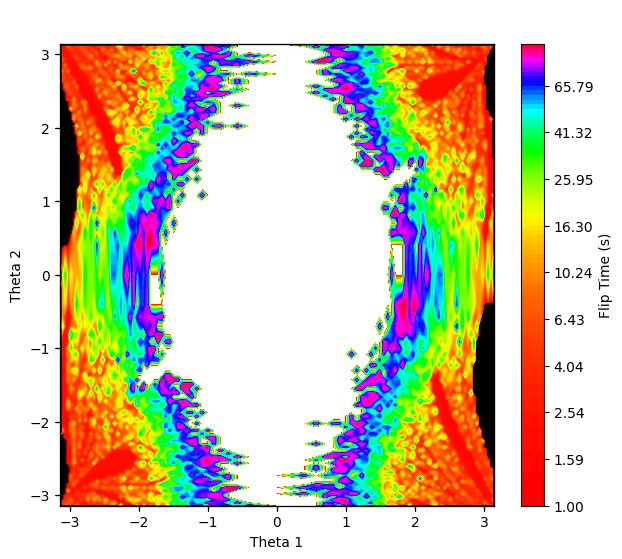
Modifying the code we can now plot a heat map (low res to save cpu) showing the time to first flip (>π rotation) of one of the pendula, for different starting combinations of the displacement angles θ1 and θ2. Areas in black correspond to < 1s to first-flip, red to magenta 1-100s (log scale). It's just one 3D projection from the multi-dimensional space that includes mass, length and momentum combinations! Area in white is the set of angles that give rise to stable swings of the pendulum, no flip or tipping point to chaos!
If only the climate was so simple!
https://www.visualcapitalist.com/countries-by-share-of-earths-surface/
https://www.nature.com/articles/33859; https://www.scientificamerican.com/article/behind-the-hockey-stick/
https://web.mit.edu/jorloff/www/chaosTalk/double-pendulum/double-pendulum-en.html
https://www.carbonbrief.org/explainer-how-the-rise-and-fall-of-co2-levels-influenced-the-ice-ages/
Figure 7.2 in IPCC, 2021: Chapter 7. In: Climate Change 2021: The Physical Science Basis. Contribution of Working Group I to the Sixth Assessment Report of the Intergovernmental Panel on Climate Change. doi: 10.1017/9781009157896.009
Dansgaard, W., Johnsen, S. J., Clausen, H. B., Dahl-Jensen, D., Gundestrup, N. S., Hammer, C. U., Hvidberg, C. S., Steffensen, J. P., Sveinbjörnsdottir, A. E., Jouzel, J., and Bond, G.: Evidence for general instability of past climate from a 250-kyr ice-core record, Nature, 364, 218–220, https://doi.org/10.1038/364218a0, 1993.
David I. Armstrong McKay et al. Exceeding 1.5°C global warming could trigger multiple climate tipping points. Science 377, eabn7950 (2022). DOI:10.1126/science.abn7950
Naughten, K.A., Holland, P.R. & De Rydt, J. Unavoidable future increase in West Antarctic ice-shelf melting over the twenty-first century. Nat. Clim. Chang. 13, 1222–1228 (2023). https://doi.org/10.1038/s41558-023-01818-x
M. A. Srokosz, H. L. Bryden. Observing the Atlantic Meridional Overturning Circulation yields a decade of inevitable surprises. Science 348, 1255575 (2015). DOI:10.1126/science.1255575
Caesar, L., McCarthy, G.D., Thornalley, D.J.R. et al. Current Atlantic Meridional Overturning Circulation weakest in last millennium. Nat. Geosci. 14, 118–120 (2021). https://doi.org/10.1038/s41561-021-00699-z
Latif, M., Sun, J., Visbeck, M. et al. Natural variability has dominated Atlantic Meridional Overturning Circulation since 1900. Nat. Clim. Chang. 12, 455–460 (2022). https://doi.org/10.1038/s41558-022-01342-4
McCarthy Gerard D. and Caesar Levke. 2023 Can we trust projections of AMOC weakening based on climate models that cannot reproduce the past? Phil. Trans. R. Soc. A. 381 2022019320220193. http://doi.org/10.1098/rsta.2022.0193
René M. van Westen et al. Physics-based early warning signal shows that AMOC is on tipping course.Sci. Adv. 10, eadk1189(2024). DOI:10.1126/sciadv.adk1189
IPCC, 2021: Climate Change 2021: The Physical Science Basis. Contribution of Working Group I to the Sixth Assessment Report of the Intergovernmental Panel on Climate Change [Masson-Delmotte, V. et al]. Cambridge University Press, Cambridge, United Kingdom and New York, NY, USA, 2391 pp. doi:10.1017/9781009157896.
Ditlevsen, P., Ditlevsen, S. Warning of a forthcoming collapse of the Atlantic meridional overturning circulation. Nat Commun 14, 4254 (2023). https://doi.org/10.1038/s41467-023-39810-w
NASA's Scientific Visualization Studio, data provided by Robert B. Schmunk (NASA/GSFC GISS). https://svs.gsfc.nasa.gov/5207/
Jackson, L.C., Biastoch, A., Buckley, M.W. et al. The evolution of the North Atlantic Meridional Overturning Circulation since 1980. Nat Rev Earth Environ 3, 241–254 (2022). https://doi.org/10.1038/s43017-022-00263-2
Fan, Y., Liu, W., Zhang, P. et al. North Atlantic Oscillation contributes to the subpolar North Atlantic cooling in the past century. Clim Dyn 61, 5199–5215 (2023). https://doi.org/10.1007/s00382-023-06847-y
Stuart A. Cunningham, Christopher D. Roberts, Eleanor Frajka-Williams, William E. Johns, Will Hobbs, Matthew D. Palmer, Darren Rayner, David A. Smeed, Gerard McCarthy. Atlantic Meridional Overturning Circulation slowdown cooled the subtropical ocean. Geophys. Res. Lett., 40, 6202–6207, doi:10.1002/2013GL058464, 2013
Orihuela-Pinto, B., England, M.H. & Taschetto, A.S. Interbasin and interhemispheric impacts of a collapsed Atlantic Overturning Circulation. Nat. Clim. Chang. 12, 558–565 (2022). https://doi.org/10.1038/s41558-022-01380-y
https://www.independent.co.uk/climate-change/news/atlantic-ocean-currents-collapse-freeze-b2494791.html; https://metro.co.uk/2024/02/12/uk-faces-day-tomorrow-style-apocalypse-ocean-current-switches-off-20265924/
https://www.telegraph.co.uk/news/2024/02/09/climate-change-modelling-wrong-claim-uk-scientists/; https://theconversation.com/the-gulf-stream-will-not-collapse-in-2025-what-the-alarmist-headlines-got-wrong-210773
https://www.bbc.co.uk/news/science-environment-66289494; https://www.forbes.com/sites/davidbressan/2022/04/12/gulf-stream-collapse-will-likely-not-cause-climate-catastrophe/?sh=1ba7d8dc4b79
https://www.southparkstudios.co.uk/video-clips/y7xyt1/south-park-randy-s-theory
*Links accessed 13/03/2024